Types of Magnetism - Magnetic Materials
Descriptions of the 5 types of magnetism in materials
There are 5 classifications of magnetism, all exhibiting unique properties. They are (in no particular order):
- Paramagnetic
- Diamagnetic
- Anti-Ferromagnetic
- Ferromagnetic
- Ferrimagnetic
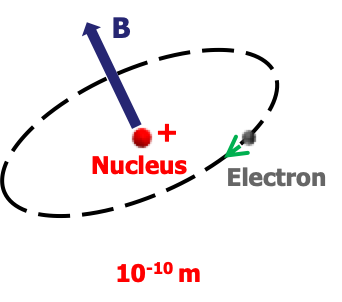
Figure 1 - Flux density (B) direction created from an electron orbiting the nucleus in an atom.
Every material falls into one of these 5 categories. Therefore, every material is technically a magnetic material. Every atom has moving electrons that create and respond to magnetic fields. We typically categorize them using their permeability and domain alignment.
It is very important to understand the concept of permeability as it pertains to magnetic materials. Think of permeability as magnetic “conductivity” and the flux lines as “current” (we call this permeability and flux in magnetic circuits, but they are direct analogs. Actually, all magnetic properties have electrical analogs, as they are two sides of the same coin). The higher the permeability, the tighter the flux lines can push together in the material (i.e., the more flux can “flow” in the material).
Paramagnetic
The paramagnetic category of magnetism is for materials that are negligibly affected by external magnetic fields. We typically call this (and a few others) category “non-magnetic”, although technically incorrect on a very small sale, these materials have no noticeable effect on the field in most applications. You can measure this very small magnetic influence in these materials with a simple experiment. Hang a paramagnetic material from a string and place it in a magnetic field. It should slowly turn to align with the field. Due to the lack of friction, the material is free to rotate from the very little torque created by the field but note that this will appear as no change given even small amounts of friction, hence the “non-magnetic” designation.
Paramagnetic materials have a relative (to vacuum space) permeability very near, but not below, 1. So, they carry flux about as well as free space (vacuum space). Air is actually a paramagnetic material. The majority of materials are paramagnetic.
Example paramagnetic materials:
- Air
- Plastic
- Brass
- 300 series stainless steel
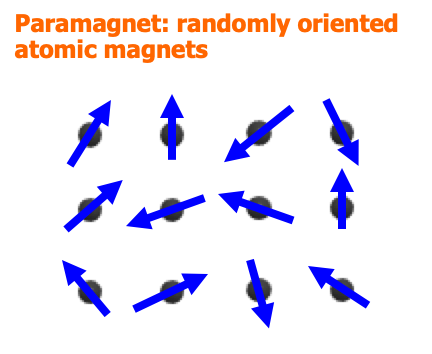
Figure 2 - Example of the domain orientations in paramagnetic materials.
Diamagnetic
Diamagnetic materials are so close to paramagnetic materials that they again fall into the category of “non-magnetic”. In a similar experiment to the one proposed in the paramagnetic section, we can hang a diamagnetic material suspended in a magnetic field and it will misalign with the field instead of align. The difference is again very small.
The relative (to vacuum space) permeability is typically very near, but not above, 1. So, they carry flux just a little worse than free space (vacuum space). Water is a great example of a diamagnetic material. The flux lines will spread out a little further than when they are in air (less total flux per area).
Technically, any material with a permeability below 1 is diamagnetic, but it is very rare that these materials exhibit permeabilities outside of the “very near 1” range. Superconductors can be closer to 0 and are still diamagnetic. They are a rare and specific circumstance where a material cannot carry flux and the flux lines will have to curve around the material entirely (the long wanted “magnetic shield”), which would be a great leap forward for magnetic circuit design if these properties were exhibited at non-supercooled temperatures.
Examples:
- Water
- Copper
- Bismuth
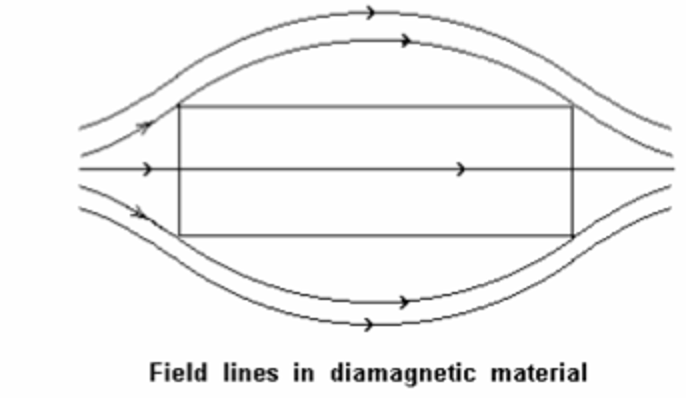
Figure 3 – Field lines spreading further apart in a diamagnetic material.
Antiferromagnetic
Antiferromagnetic materials are a very interesting category. They are closely related to paramagnetic materials (another “non-magnetic” category, because the effects are so small. They are oriented, unlike paramagnetic materials, but are perfectly balanced so that they do not affect the field around them. They can become paramagnetic above certain temperatures (randomly orienting the domains).
These materials are VERY rarely used in normal permanent magnet design applications. Mostly in physics experiments and small-sized memory applications (hard drive heads).
Examples:
- Chromium
- Hematite
- Iron-Manganese
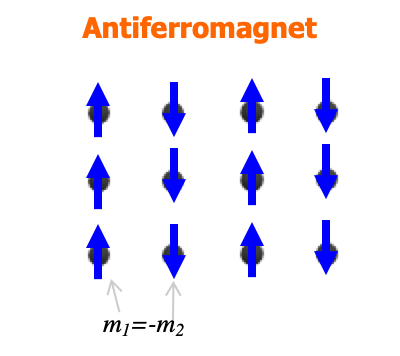
Figure 4 – Example of the domain orientations in antiferromagnetic materials.
Ferromagnetic
Ferromagnetism is the category we traditionally think of when we call something magnetic. These materials greatly affect the magnetic field and can even induce the field. Most of the materials we use in permanent magnet design (both hard and soft magnets, sintered NdFeB and steel, our two most common tools) fall into this category.
Typically, these are composed of a ferromagnetic transition metal compound. So, think iron, nickel, or cobalt mixture. The relative permeability of ferromagnetic materials ranges from near 1 (not as close to very near 1 as paramagnetic materials) to extremely high (200,000 or even 1,000,000). These high permeability metals are to magnetic circuits what copper is to electrical circuits. As copper is “low resistance” as a conductor of electrical current these materials are “low reluctance” as a conductor of magnetic flux.
Permanent magnets typically fall into the low permeability side of ferromagnetism. While they are terrible “carriers” or conductors of flux (relative permeability near 1, 1.05-ish range) while unmagnetized, they have the ability to induce a field (they can create a magnetic field around them) once magnetized. When first mixed, pressed, and sintered, their magnetic domains are perfectly misaligned (along a single axis in the case of anisotropic materials like sintered NdFeB and sintered SmCo), balanced against each other so there is no external field present. This is the unmagnetized state. A large magnetic field produced around them by an outside influence (a magnetizer) can align all the magnetic domains in the same direction. This stacked effect of all the ferromagnetic domains pointing the same way can produce an external magnetic field, creating a permanent magnet. The domains can misalign again with increased external opposing fields or increased temperature. If this is done using temperature and they return to the perfectly misaligned state, we are said to have reached the “curie temperature” of the material (380 or so degrees C for sintered NdFeB). This starts to happen at a much lower temperature than the curie temperature based on the shape and coercivity of the material (partial demagnetization).
Examples:
- Neodymium-Iron-Boron Magnets (Neo magnets)
- Iron
- Steel
- Iron-Cobalt
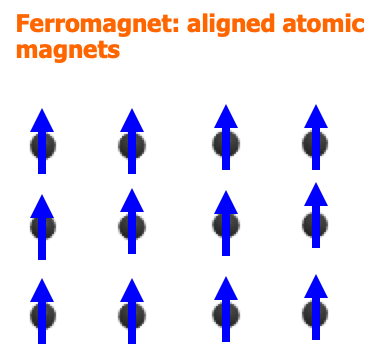
Figure 5 – Example of the domain orientations in ferromagnetic materials.
Ferrimagnetic
Ferrimagnetic materials are typically lumped into the ferromagnetic category, because in application they are not that different. The defining difference is the alignment of the magnetic domains. In ferromagnetic materials, the domains all can be aligned under a magnetic field. In ferrimagnetic materials there remains some slight opposing alignment internally. Most of the strong domains will align in the same direction, working together. There will remain some weaker domains pushing the other way, negating some of the material’s full potential. This is also similar to antiferromagnetism, except the aligned and misaligned are NOT balanced.
The relative permeability of ferrimagnetic materials is the same as the ferromagnetic category. Anywhere from near 1 to extremely large. Many ferromagnetic materials can become ferrimagnetic as they interact with their environment through chemical reactions; this is often an unwanted change. The ferromagnetic material will be plated (or coated) in such a way to isolate it from these changes (coating in an inert metal or other options to prevent oxidation or environmental reactions).
Examples:
- Magnetite (iron-oxides… rust)
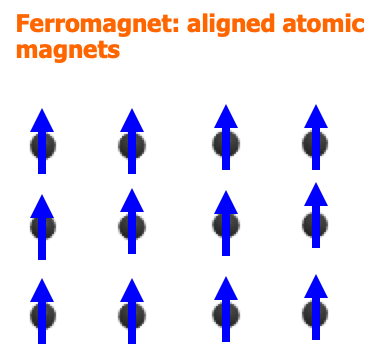
Figure 6 – Example of the domain orientations in ferrimagnetic materials.